Resolving electronic gridlock
in a Mott insulator
Scientists from the Cavendish’s Quantum Matter group have studied how a material known as a Mott insulator transforms into a metal under extreme pressures, giving insights into how their electrons go from gridlock to freedom.
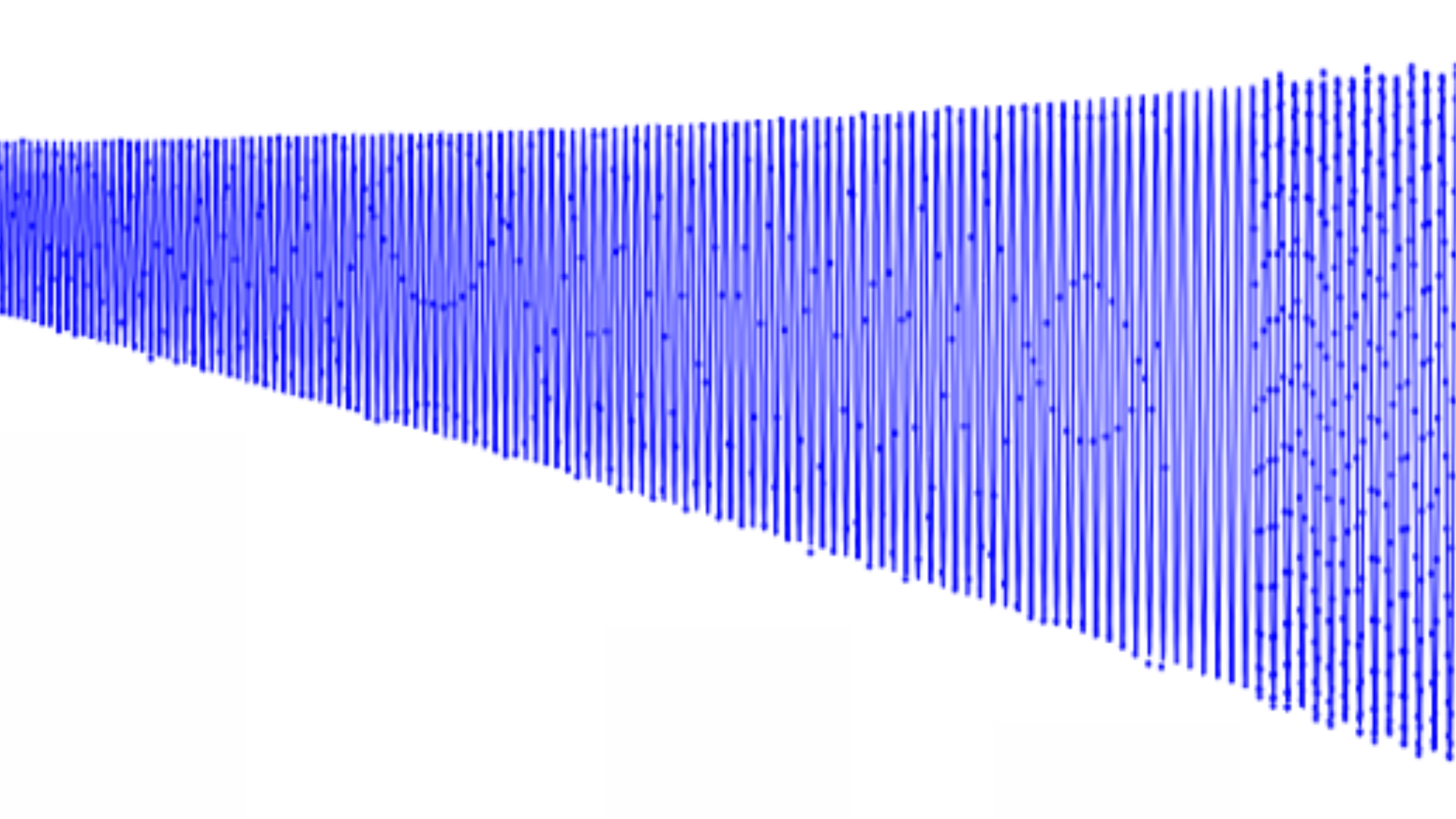
Mott insulators do not lack conduction electrons as is the case in a conventional insulator or semiconductor. Instead, electrons are locked into position by their mutual interaction. Tuning a Mott insulator by varying the distance between the atoms in the lattice under applied pressure shifts the balance between epulsive interaction and the tendency of electrons to spread out. This makes it possible to turn a Mott insulator into a metal and study the correlated metallic state, in which the grid-locked electrons have been set free.
Comparison between a conventional band insulator and an interaction-induced Mott insulator.
Comparison between a conventional band insulator and an interaction-induced Mott insulator.
Foremost among the scientific questions surrounding this ‘Mott metal’ state on the threshold of ‘Mott localisation’ (the point at which conduction electrons become locked in place) is the velocity distribution of the conduction electrons: because the electrons are always in motion, we do not determine their position but rather examine the relationship between their momentum and energy by observing the electronic Fermi surface and effective mass. The Fermi surface separates filled from unfilled electronic states in momentum space at low temperatures. Without the lattice atoms, it would be spherical, but in real materials it can have a more intricate shape. The electronic effective mass can in a real material be orders of magnitude different from the mass of free electrons in vacuum. It is strongly affected both by the interaction with lattice atoms and by interactions between the electrons themselves. The effective mass tells us how fast the electrons in momentum states near the Fermi surface are moving.
Observing quantum oscillatory phenomena in high magnetic fields and at low temperatures provides direct access to these key properties, using a set of techniques that was pioneered at the Cavendish by David Shoenberg. To measure the mass of electrons in vacuum, JJ Thomson observed the deflection of the electrons’ trajectories in a moderate applied magnetic field. A similar phenomenon, the Hall effect, exists for electrons moving in a metal, but it does not allow precise measurements of the effective electron mass. Quantum oscillations, by contrast, are associated with the closed cyclotron orbits of conduction electrons in very high magnetic fields, when the electrons spiral around the magnetic field lines. Only certain orbits areallowed by quantum mechanics, and this quantisation depends on the strength of the applied field. Many material properties oscillate with applied field, as subsequent orbits in momentum space pass through key sections of the electronic Fermi surface. At the time of Shoenberg, quantum oscillations could rarely be observed in anything but the cleanest elemental metals. The far higher magnetic fields available today have dramatically widened the applicability of this method, which my Quantum Matter group colleagues have recently applied to resolve the electronic structure of the novel superconductor UTe2.
Quantum oscillations observed in the pressure-metallised Mott insulator NiS2 (top panel) indicate little change in the Fermi surface size with pressure (bottom left), whereas the effective mass of the electron charge carriers rises rapidly, as the insulating state is approached (bottom centre). Measurements employed a radio-frequency tank circuit technique with pickup coil inside the high-pressure sample space (bottom right).
Quantum oscillations observed in the pressure-metallised Mott insulator NiS2 (top panel) indicate little change in the Fermi surface size with pressure (bottom left), whereas the effective mass of the electron charge carriers rises rapidly, as the insulating state is approached (bottom centre). Measurements employed a radio-frequency tank circuit technique with pickup coil inside the high-pressure sample space (bottom right).
High precision measurements under extreme hydrostatic pressures generated in miniature gemstone anvil pressure cells are required in order to record quantum oscillations in a pressuremetallised Mott insulator. We have overcome this challenge by tracking changes at the part-per-10-million level of the resonance frequency of a tank circuit oscillator, which probes sample properties via the effect they have on the self-inductance of a small inductor placed within the high-pressure sample volume. Using this novel technique, Quantum Matter group scientists and colleagues at high field facilities in the USA and the Netherlands have resolved the electronic structure in high-purity samples of the Mott insulator NiS2 at sub-Kelvin temperatures, applied magnetic fields of up to 35 T and at pressures reaching beyond 100,000 atmospheres.
Our results show that as the threshold of Mott localisation is approached from the metallic side by reducing the pressure, the electrons appear to grow heavy and they slow down dramatically, but their concentration hardly changes. In a ‘Mott metal’, interactions boost the effective electron mass – a phenomenon that generalises to more complex systems such as cuprate, organic and iron-based superconductors, Moiré superlattice systems and Kondo lattice materials. These materials display numerous collective phenomena of current interest, such as various distinct types of superconductivity and magnetism as well as other, more subtle forms of order. Our understanding and, ultimately, the ability to manipulate these phenomena depend on resolving the nature of the underlying correlated metallic states from which they arise. Our high-pressure study in NiS2 shows how this may be achieved.
A. G. Eaton et al., 'Quasi-2D Fermi surface in the anomalous superconductor UTe2',Nature Communications, 15, 223 (2024).
K. Semeniuk et al., 'Truncated mass divergence in a Mott metal', Proc. Natl. Acad. Sci. 120, e2301456120 (2023).