The oldest black hole
An international team led by astronomers at the Cavendish Laboratory have discovered a primeval black hole that existed over 13 billion years ago during the era known as cosmic dawn. Its discovery is challenging our understanding of how black holes form.
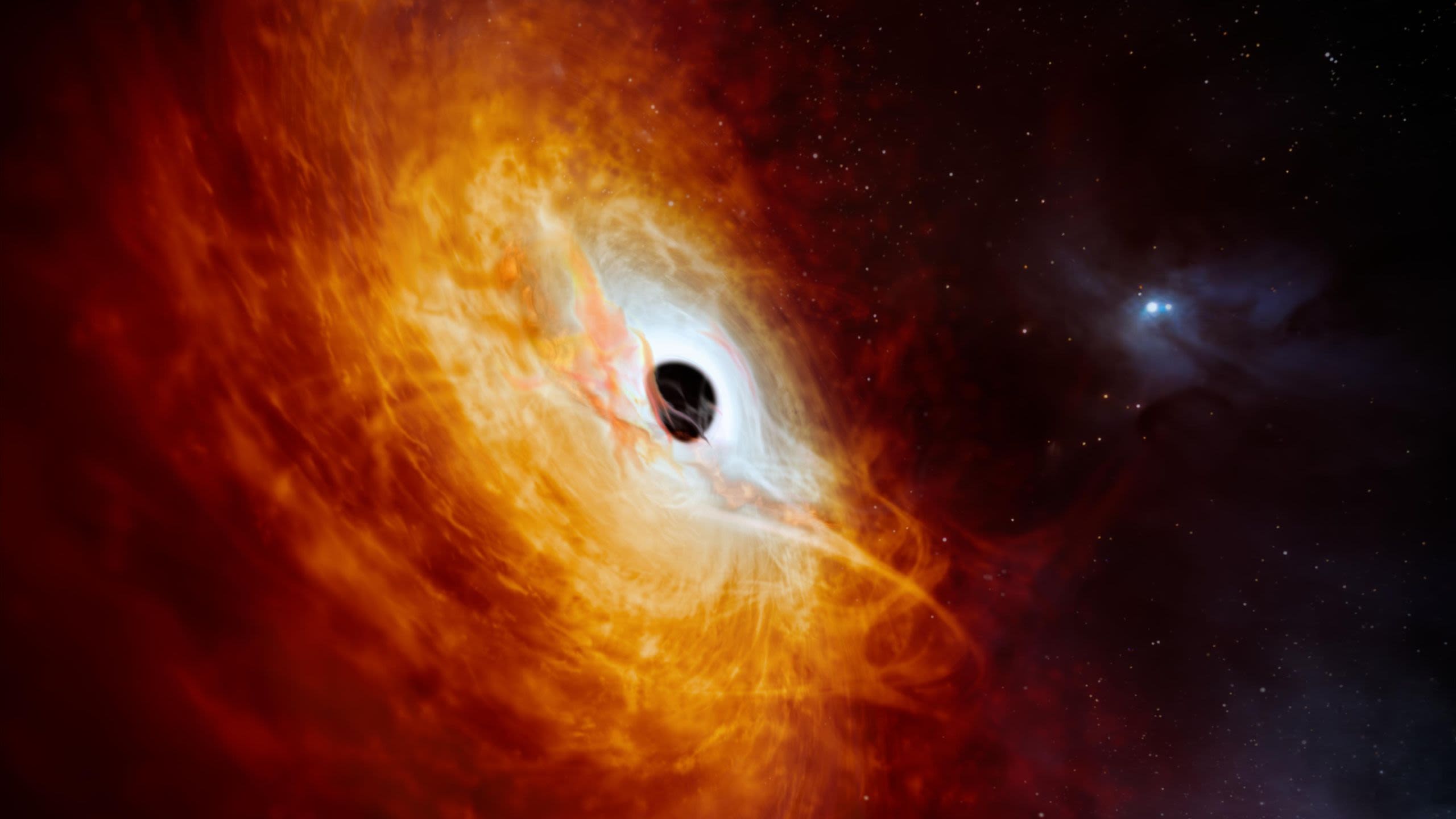
Revolutionary. This is one of the most frequently used adjectives in the plethora of papers that have been published with data from the James Webb Space Telescope so far. This is no overstatement. Webb is truly revolutionising many areas of astrophysics. With its 6.6-metre mirror making it the largest telescope in space, Webb’s sensitivity in some infrared bands is up to a thousand times better than any previous instrument. Such an enormous leap in sensitivity has only been achieved a handful of times in the history of astronomy, or indeed in the whole of science. To give a sense of the magnitude of the improvement, it’s equivalent to instantaneously upgrading Galileo’s four-centimetre telescope to modern ten-metre telescope! It is therefore not surprising that Webb is opening spectacular new vistas on the cosmos. The exploration of infant black holes in the early universe is one area whose horizon is drastically expanding thanks to the advent of Webb.
We know that most galaxies in the local universe have supermassive black holes at their centres. These giants have masses ranging from millions to billions of times that of the Sun, and account for between 0.1% to 0.5% of the total mass of their host galaxy. At the centre of our own Milky Way is a black hole with a mass of four million suns, whose discovery was rewarded with Nobel prizes for Andrea Ghez and Reinhard Genzel in 2020.
How these supermassive black holes came to be is one of the most intriguing questions in astrophysics. The standard scenario is that their first seeds were the remnants of massive stars that died in supernova explosions, leaving black holes with masses of a few tens, or possibly a few hundred suns. By accreting matter from the surrounding environment these black holes then grew to much larger sizes.
However, there is a theoretical maximum rate at which black holes can grow. Black holes typically consume gas from a so-called ‘accretion disc’, which becomes extremely hot (around 100,000 degrees) and radiates intense ultraviolet light. If the accretion rate is too high, then the pressure of this radiation on infalling matter overcomes the black hole’s gravitational pull and prevents it from growing any faster. This is the so-called ‘Eddington limit’.
To test this theory, we need to look back in time to observe the first phases of black hole formation and growth in the early universe. Webb can act as just such a time machine. Its unprecedented sensitivity allows it to spy extremely distant (and hence faint) galaxies that, due to the finite speed of light, are seen as they were in the remote past, up to several billion years ago.
Webb has discovered dozens of new black holes in their accretion phase, dating back more than 10 billion years. My own team at the Cavendish recently discovered the most distant ever of these black holes using NIRSpec, the primary spectrometer aboard Webb. We found the black hole in the core of a galaxy known as GN-z11, which dates back more than 13 billion years to when the universe was a mere 440 million years old, young in cosmic terms. This early epoch is known as ‘cosmic dawn’, the time when the first stars burst into light.
The black hole was identified through multiple signatures in the electromagnetic spectrum produced by its host galaxy. Specifically, it was possible to unambiguously identify the presence of extremely dense gas, tell-tale atomic transitions, and powerful winds of ionised gas with speeds of around one thousand kilometres per second, all typical of accreting supermassive black holes.
This primeval black hole is estimated to weigh in at around 2 million solar masses, making it relatively small compared to the gargantuan black holes found in later epochs, many of which have masses of over a billion suns. However, the black hole in GN-z11 is nonetheless surprisingly large given the relative youth of the universe during that epoch. In fact, there should not have been enough time so early in the universe for the black hole to grow to such a vast size through the standard mechanisms that we know of in astrophysics.
Black hole mass as a function of age of the universe and redshift.
Black hole mass as a function of age of the universe and redshift.
This is illustrated in the figure above showing the black hole mass (on the vertical axis) as a function of age of the universe (upper horizontal axis). The lower horizontal axis shows how the age of the universe translates into ‘redshift’, a measure of how much the wavelength of light is stretched while travelling to us due to the universe’s expansion. The golden circle shows the mass of the black hole in GN-z11 and the blue shaded region shows its mass projected back in time assuming that it was accreting steadily at the Eddington limit. Crucially, this shows that even if the black hole had grown as fast as theoretically possible, to reach its observed size it would have needed to have had a mass of over a thousand suns just 100 million years after the Big Bang. Even assuming that it formed within the first few tens million years after the Big Bang (which is extremely unlikely since few stars are thought to have formed that early) it is extremely challenging to explain the black hole in GN-z11 as a stellar remnant uninterruptedly accreting at the Eddington limit.
This result has prompted astrophysicists to explore new scenarios. Some have proposed that the seeds of early black holes were born ‘big’ with masses of a few hundred thousand times the mass of the Sun (known as ‘heavy seeds’), via the direct gravitational collapse of massive, pristine clouds of gas. Others have suggested that dense clusters of black holes and stars may have undergone a runaway merging process that rapidly produced intermediate mass black holes, with masses of tens of thousands times the mass of the Sun (‘intermediate mass seeds’), which then accreted matter to reach the mass seen in GN-z11. Yet another possibility is that the black hole seeds are stellar remnants (‘light seeds’), but they manage to gobble matter very voraciously, above the Eddington limit, even if only in short bursts.
As illustrated in the figure, all these scenarios can reproduce the large black hole mass observed in GN-z11. However, it is particularly intriguing that the black hole itself is found to be accreting at five times the Eddington limit. This supports the idea that the super-Eddington scenario is actually a realistic possibility. Subsequent work by my team, in particular by Cavendish PhD student and P.C. Ho Scholar Ignas Juodžbalis, produced additional evidence that this is a likely route for the early formation and growth of black holes.
One possible explanation for this extremely rapid rate of accretion is that the infalling matter is self-shielding from the strong radiation pressure. However, it is expected that such phases of extremely vigorous accretion cannot be sustained for long periods and must be short lived. In between these voracious meals most of these infant black holes must spend much of their early life in a dormant state.
“This slew of exciting discoveries is just a first glimpse of the results that will inevitably flow from the James Webb Space Telescope”
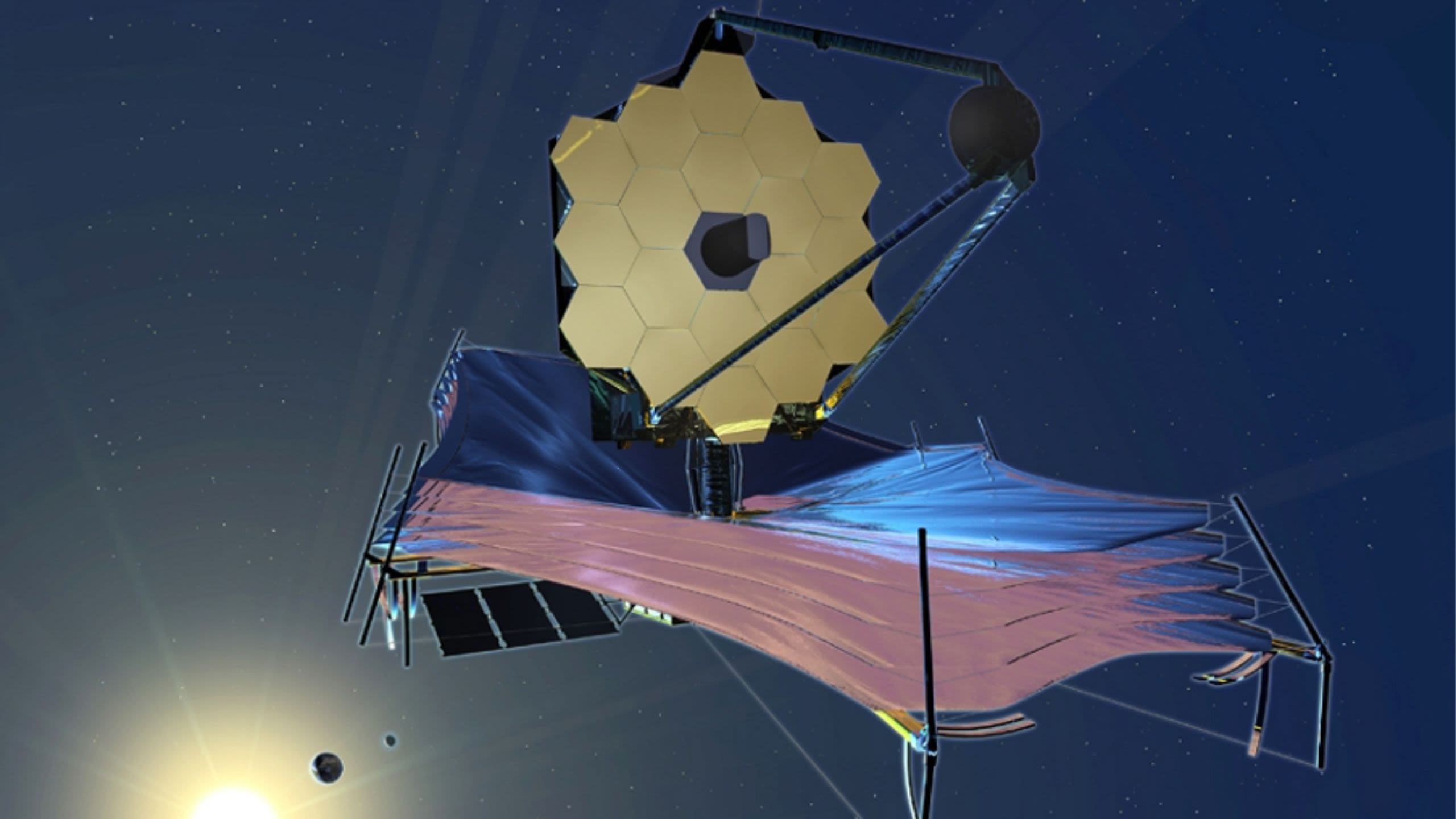
Another interesting feature of the black hole in GN-z11 and many other infant black holes recently discovered by Webb, is that they are ‘overmassive’ relative to their host galaxies. In contrast to local, mature black holes, their infant counterparts in the distant universe can have masses that are comparable to their host galaxies! While nature appears to have facilitated the rapid growth of black holes, the formation of stars in their host galaxies lagged behind, perhaps inhibited by the large quantities of energy released by their matter-guzzling black holes.
Yet another exciting result that we obtained from Webb is the discovery of a growing number of supermassive black hole pairs in the early universe. The most distant and spectacular of these was found by Newton-Kavli Junior Fellow Hannah Übler in a galaxy that existed 750 million years after the Big Bang. These results add yet another unexpected dimension to the problem and suggest that supermassive black hole mergers are another important route for their rapid growth in the early universe. These violent collisions would have produced gravitational waves that will be detectable by the next generation of gravitational wave observatories, such as the LISA space mission recently approved by the European Space Agency.
This slew of exciting discoveries is just a first glimpse of the results that will inevitably flow from the James Webb Space Telescope, coming from just its first year of operation. This revolutionary instrument is expected to operate for another twenty years. The most exciting discoveries are undoubtedly yet to come!
Roberto Maiolino is Professor of Experimental Astrophysics at the Cavendish Laboratory and the Kavli Institute for Cosmology, Cambridge.
R. Maiolino et al., 'A small and vigorous black hole in the early Universe', Nature, 627, 59 (2024).